Colonies in Space:
Chapter 15 – Ring Around the Sun Chapter 15 – Ring Around the Sun
Colonies in Space
by T. A. Heppenheimer
Copyright 1977, 2007 by T. A. Heppenheimer, reproduced with permission
Chapter 15 – Ring Around the Sun
The moon glows softly in the night sky. Lovers admire it, poets sing songs to it, scientists contemplate its resources. But for a long-term source of materials for space colonization, it may be we will look, not to the moon, but to the asteroids. The asteroids are a collection of small bodies, located mostly between the orbits of Mars and Jupiter, with some crossing the orbit of Earth. They form a diverse population, rich in resources and in convenient size and location for human use for the centuries to come.
Today about 2000 are known well enough that they can be observed at will and a number of others have been observed sporadically. In all there are probably about 100,000 which could be seen with our largest telescopes. There is a continual gradation from large asteroids to small ones, to still smaller chunks of rock and down to the finest particles of dust.
However, they are not remnants of a shattered planet, nor are most of them in any way connected with meteorites. Superman’s planet Krypton notwithstanding, planets don’t just fragment or shatter, since they are held together by gravity. Asteroids today are regarded as material left over from the formation of the solar system, material which failed to coagulate into a planet. The asteroids are mostly in very stable orbits which stay far away from other planets; so chunks of rock which are knocked loose have no way to get to Earth.
Some common types of meteorites are also of great interest to the future of space colonization. There are iron and stony-iron meteorites, in many of which the metal is alloyed with nickel, ready for extraction by melting.
There are various types known as “chondrites,” because they contain small inclusions known as “chondrules.” These are more nearly similar to the primitive material from which planets were formed, in which iron was not separated out as if to make it ready for the rolling mill. Ordinary chondrites are about one-tenth iron; better still, they have up to 0.3 percent water. Even better in this respect are the meteorites of the most primitive composition, the “carbonaceous chondrites.” These contain up to several percent carbon, which makes them very dark in color. Some of this carbon is in organic compounds; so carbonaceous chondrites typically have about half the organic content of oil shale. Best of all, they may have up to 20 percent water. They also have up to 0.04 percent nitrogen—not a lot, but worth having.
The metal content of these meteorites differs from the moon. They typically have only one-tenth the percentage of aluminum and less than 10 percent the titanium. But they are several times richer in magnesium and there is no lack of iron.
All these results are from laboratory studies on meteorites, and it is a big jump from the study of a 6-inch specimen 2 feet away to study of a 100-mile diameter asteroid, 100 million miles away. But through some rather startling advances in observational techniques, it is now possible for an astronomer to prospect for desired materials by working at the telescope, with better assurance of what he will find than if he were a terrestrial prospector with a mule and pickax—or, for that matter, with a spacecraft for multispectral photography of Earth.
Astronomers have long used the technique of measuring the apparent brightness of an object at two or three wavelengths and then comparing the measurements to obtain useful information. In 1968, Tom McCord of MIT devised a convenient means of measuring the apparent brightness not at 2 or 3, but at 24 wavelengths, giving a spectrum. He first used this instrument to study the asteroid Vesta, working with his associates John Adams and Torrence Johnson. To their surprise, repeated observations gave a spectrum which matched almost precisely a moon-rock spectrum which Johnson had on his desk. The surface of Vesta was made of the volcanic rock basalt—Vesta’ s surface had once been molten lava.
In 1969, an MIT graduate student, Clark Chapman, was looking for a topic for his dissertation. McCord suggested he survey the spectra of asteroids with his instrument, which he did. It took him two or three years because he had to use bits and pieces of time at the telescope, left for him by more senior astronomers. But he got both his data and his Ph.D. His data showed mainly that there were different types of spectra, few of which could be identified by comparison with meteorites or lunar samples.
Back in the laboratory Mike Gaffey at MIT did studies on the spectra of meteorite samples. In the meantime, other people were finding some very significant additional pieces to the puzzle. At Cornell, Joe Veverka was working on the problem of determining what fraction of sunlight is reflected by an asteroid, what astronomers call its albedo. He found a way to determine this by studying the degree to which the reflected light is polarized, as if it had passed through Polaroid glass. At Caltech, Dennis Matson was working on an entirely separate means of determining albedo from observations of asteroids in the infrared. One of the first asteroids he observed was Bamberga, number 324. He found its albedo was 0.03, meaning it reflects only 3 percent of the sunlight shining on it.
This meant Bamberga is one of the darkest objects in the solar system—darker than a blackboard, nearly as dark as soot from a fire. Only one thing could make Bamberga so black—carbon. Bamberga looked as if it might be a carbonaceous chondrite.
By 1972 it was possible to directly measure a number of important properties of asteroids and compare them with laboratory samples. By then, Clark Chapman had gone to Arizona, where it was much easier for him to get observing time on the big telescopes; Tom McCord stayed at MIT, along with Mike Gaffey. By mid-1974 these last two were able to write a paper for Science setting forth the basic rules for determining the composition of the surface of asteroids from the observed data, as well as giving the surface composition for fourteen asteroids. Ceres was found to be another carbonaceous chondrite, at least on the surface, as was Pallas. However, Juno looked like a stony-iron meteorite. The large asteroid Psyche, number 16, appeared to be a mass of nearly pure iron—not any iron, but quite likely the best grade of nickel steel.
Meanwhile Chapman and his associates were studying dozens of asteroids. At about the same time they announced an important general discovery: 90 percent of the main-belt asteroids fall into two categories. The first and larger group is similar to carbonaceous chondrites. The second class resembles stony-iron meteorites. Chapman called these classes C-type and S-type. But the asteroids whose orbits cross that of the earth or of Mars, were found in many cases to resemble a third type: ordinary chondrites.
All these studies represented work in astronomy by people who were entirely unaware of space colonization. It was Eric Drexler who pointed out the connection, in conversations with Mike Gaffey. If asteroidal resources were to be used to colonize space, there still remained a problem. As a source of raw materials the moon is close at hand and easy to reach. Most of the asteroids are several hundred times more distant. What’s more, most asteroids’ orbits are not nearly circular but are markedly elliptical, which makes them harder to reach. Worse, the orbits are tilted or inclined with respect to Earth’s orbit, which makes reaching them even harder. This meant that mining the asteroids would be much more difficult than mining the moon, requiring far more advanced propulsion as well as extensive capabilities for supporting large crews for a long time in interplanetary flight. If space colonization using lunar resources looked like something that in a natural way could grow out of near-term efforts on lunar bases, large space stations, and space construction, then using asteroidal resources looked like something that would be several decades further off. After all, we had landed astronauts on the moon but have yet to send even a simple flyby mission to an asteroid.
Eugene Shoemaker came to the rescue with the discovery of 1976 AA. When Gene Shoemaker got his Ph.D in geology late in the 1940s, he decided that someday geologists would be able to study the moon at close quarters and that it would happen in his lifetime. He couldn’t talk much about such wild notions with his colleagues; so he made the acquaintance of the few lunar astronomers, like Gerard Kuiper, who were engaged in what was then a lonely and neglected branch of astronomy. He kept at this all through the 1950s, much as Gerry O’Neill later would do with his private dreams of space colonies. Then came Sputnik and Shoemaker found he had other people to talk to.
It fell to Shoemaker to work out the major geologic ages or epochs in the history of the moon. On Earth in geologic history we have the Cretaceous and Permian and Silurian epochs. On the moon there are the Imbrian, the Procellarian, the Eratosthenian and Copernican—all named for major craters or maria.
In 1969 Shoemaker was picked as chairman of Caltech’s Division of Geological and Planetary Sciences. But he found his administrative duties interfered with the work he loved; so he resigned the chairmanship after a couple of years and went back to doing research. What interested him now was not the moon but the asteroids.
After all, craters like those of the moon come about when a small asteroid, or a chunk of one, finds itself on an orbit which intersects that of Earth. He set up a program to search for these asteroids, with Eleanor “Glo” Helin doing the observing at Palomar. Their work led them to conclude that there should be 1000 to 2000 such asteroids, with diameters greater than a kilometer. This was a far larger number than previously proposed, making it appear that the “Earth-crossing asteroids” could be the long-sought principal source of the earth’s meteors.
Then on January 7, 1976, “Glo” Helin observed a new asteroid about two miles in diameter. Since it was the first to be found in the new year, it was named 1976 AA. After it had been observed for several nights, there was enough data to compute its orbit, and to everyone’s surprise, its orbit was closer to the earth’s than that of any other known body except the moon. It circles the sun once every 347 days and for every 20 times Earth goes round, 1976 AA goes round 21 times. Its orbit ranges from 73 million to 106 million miles from the sun, compared to Earth’s average of 93 million. In announcing the discovery, Shoemaker said: “Aside from the moon, this asteroid is one of the easiest places to get to in our solar system.” When observed by telescope, it appeared to have the characteristics of an ordinary chondrite.
The only problem was its orbit, which was inclined to Earth’s by 19 degrees. This would make it harder to reach than we would like. However, 1976 AA is only the thirty-fifth Earth-crosser discovered, out of the total population of 1000-2000; so there is opportunity to discover other small asteroids with orbits even closer to Earth’s. What is wanted is an object with an orbit like that of 1976 AA, but with low inclination. Such an object could be reached with no more difficulty than is involved in going to the moon. Moreover, its small size would make landing on it like docking with another spacecraft. And while most such asteroids appear to be ordinary chondrites, there is no reason to doubt that some would be carbonaceous chondrites, which have more water and more carbon. The asteroid Betulia (number 1580), which nearly crosses Earth’s orbit, is of this type. Nor are such asteroids small, since a one kilometer diameter object has a mass of several billion tons.
The future of space colonization rests not only with specialists in propulsion and in other aerospace disciplines, it also rests with astronomers who will be searching for new Earth-crossing asteroids, which will be the first goals for expeditions beyond the moon. These will provide the much desired intermediate steps between manned lunar flight and manned flight deep into interplanetary space. The first steps will be only slightly more difficult than the flights which will then be in progress to the moon; yet they will begin the process of opening up the entire solar system to human settlement. As our astronauts prove out their techniques and develop their skills, they will push the space frontier deeper and deeper into the asteroids, until this frontier forms an ever widening ring around the sun.
At first, these small asteroids will be hauled and tugged into the vicinity of Earth to provide carbon and nitrogen and hydrogen for the growing colonies. For this the colonies will build additional mass-drivers, similar to the one proposed for the moon, but designed for use as rocket motors to produce thrust. These will be maneuvered out to an asteroid of interest and used to move it. Or a chemical plant may be built, running on solar energy obtained with a large mirror, and sent to the asteroid. If the asteroid is a carbonaceous chondrite, a chemical plant can extract the organic material for shipment.
We may see the day when there are installations closely resembling oil refineries on the small C-type asteroids whose orbits are near that of the Earth. Carbonaceous chondrites typically have about half the organic-chemical content of oil shale. Most of this is a complex mixture of heavy hydrocarbons, including waxes or heavy oils as well as compounds related to benzene. The asteroids may contain a wealth of petroleum beyond the dreams of the richest Arabian sheik and one day it may be feasible to provide this to Earth.
However, the main activities at the refinery will be to extract water and to process the hydrocarbons into chemical fertilizers or plastics for use back at the colony. Nitrogen compounds will also be extracted and some of these will have a rather interesting nature, since they will be purines and pyramidines—nitrogenous bases such as adenine and guanine. These are the elements of the genetic code, when found in the DNA molecule, but they are also produced by nonbiologic processes and have been found in carbonaceous chondrites.
Some of the water may be electrolyzed to hydrogen and oxygen, providing rocket fuel for the shipment. Some of the plastics may be thinly coated with metal and formed into immense gossamer solar sails to use the pressure of the solar wind and sunlight for propulsion. The sail-borne ocean commerce of centuries past, the “argosies of magic sails” of Tennyson, may find their counterparts in fleets of solar sailships which undertake the long voyage to and from those distant outposts of commerce, visible only as streaks on a photographic plate.
In the long run, this will be seen as the first step toward removing from Earth much of the unpleasant or polluting industry and shifting it instead to an industrial base which is celestial rather than terrestrial. Nor will such a resource base face the problem of limits to growth. With the entire assemblage of asteroids available, limits are absurdly high: enough to support a human population some 20,000 times larger than exists today.
But in the short run—in the first few decades of space colonization—these resources will be useful mainly in stimulating two new trends in the growth of the colonies. There will be a trend toward building colonies of the largest size and another trend toward the evolution and growth of new or specialized communities, distinct from the main colonies.
The trend toward the largest colonies would carry out the initial plans put forth by Gerry O’Neill when he first started studying space colonies in 1969. The maximum size would be set by the strength of steel cables used in construction and the largest colonies would be strengthened with cables very much like those used in building suspension bridges. Today it is a matter for surprise to see how much larger these colonies would be than the Golden Gate or George Washington bridges. The time will come when the surprise and astonishment will be reversed. Future generations will be quite accustomed to the size of structures which can be built in space but they will regard with wonder and admiration the great bridges and skyscrapers of Earth, which had to be built in gravity.
The largest colonies would be twin cylinders, each four miles across and twenty miles long. They would rotate slowly in opposite directions once every two minutes to provide normal gravity. Being linked together, they will form a unit with no net spin, which can always be pointed toward the sun. The interior of the cylinders would be divided into long strips down their length: three immense window panels of glass, alternating with three strips of land.
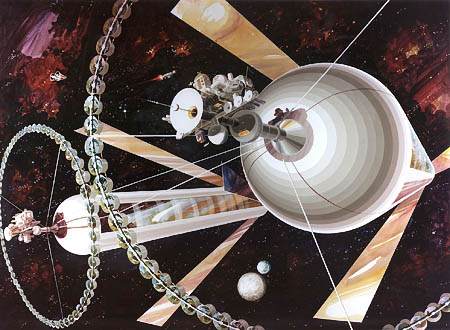
Space colony of the largest size. Each cylinder is four miles across and over twenty miles long. Manufacturing facilities are on the forward ends, and rings of cylinders for agriculture surround each colony. (Courtesy NASA) [larger image]
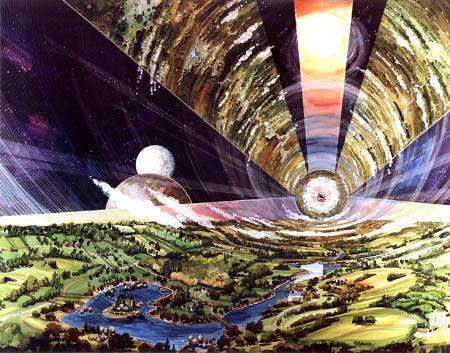
Interior of one of the large colonies, showing emphasis on parks and lakes which is possible with such huge areas (hundreds of square miles) to work with. (Courtesy NASA) [larger image]
There would be large mirrors outside to reflect sunlight through the window panels onto the land areas opposite. They would extend and retract to give night and day. Each cylinder could be surrounded by a ring of small units in which would be carried out the agriculture and manufacturing operations and there could be large solar mirrors and generating plants on the ends of the cylinders facing the sun. Inside these new worlds, clouds would form at the 3000-foot level in a blue sky—it takes 2 miles of atmosphere to make a sky blue. That atmosphere together with the soil in the land areas would be thick enough to protect against cosmic rays while the sheer size of the colony would protect against danger from meteorites. The window areas would be divided into small panels by the strengthening cables, distant and barely visible, like suspension-bridge cables which appear as thin strands of silver when seen from several miles away. If a panel were broken by a meteorite, it would take 300 years for the air to leak away.
Such colonies, if populated to the density of the Stanford torus, would be home for 20 million people. But in fact there is no need for that many. The earliest colonies will be strongly tied to Earth and governed by considerations of economics. They will resemble the early English settlements in America, founded by gentlemen who were certainly excited by the prospects they saw—but who also expected to receive dividends from the East India Company. Those early space colonies will suffice to win our initial settlements in space and build enough power satellites to solve the energy problem. By the time they are ready to build the largest colonies, they will have the resource base and stable society which will make them largely independent of Earth. The large colonies will not be akin to the early English settlements. They will much more nearly resemble the American West, with its vast promise for those who would win its land.
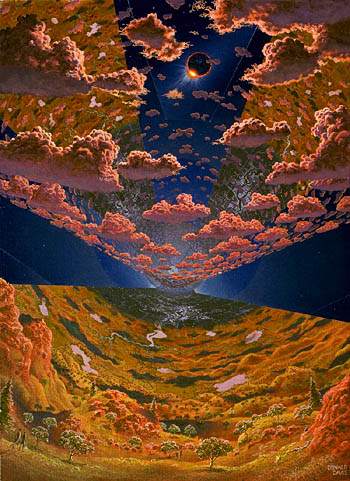
Solar eclipse inside a colony. The shadow of the earth is advancing up the valley; lights of towns are seen near the far end. (Donald E. Davis painting courtesy NASA) [larger image]
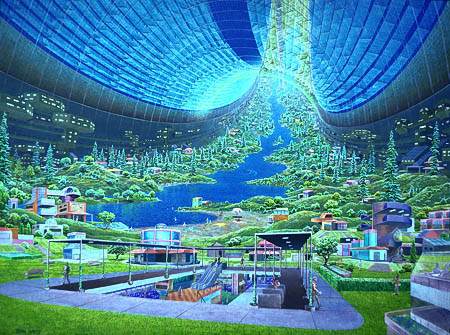
As the large colonies proliferate, the early Stanford toruses will still represent valuable living space. But their interiors will be rebuilt to suit the open, well-forested styles in vogue in the middle of the next century. (Donald E. Davis painting courtesy NASA) [larger image]
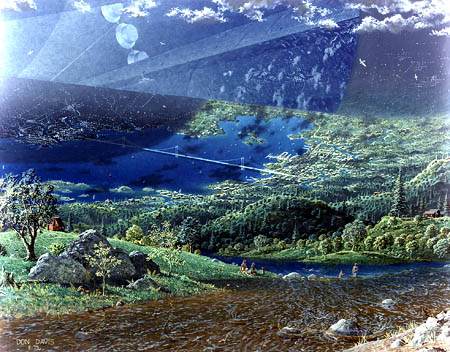
Space colony founded by a group of expatriate San Franciscans. (Donald E. Davis painting courtesy NASA) [larger image]
With cheap easy access to the resources of the moon and asteroids and space travel as routine as airline traffic is today, the largest colonies need not meet the same economic criteria as the Stanford torus. They can be designed as open, widely spread communities with comfort and beauty the main considerations. They may never be as open as the old West, with its half-dozen (or fewer) people per square mile, but their populations can easily be made one-hundredth as dense as the Stanford torus, approximating the layout of places like Columbia, Maryland, with its small communities of two-story single-family dwellings interspersed amid streams, forests and winding roads. The colonies’ forests will not grow up overnight, even in the favorable conditions for agriculture possible in the colonies, but in time they will grow.
What will people do there? Some will be the agricultural chemists, the engineers and technical workers. They will be responsible for running the colony systems and for working with the production and manufacturing plants. Others will fill the hundreds of jobs which go to make up any community—storekeepers, plumbers, TV repairmen. With automated equipment to do most routine chores, few people will be unable to choose work which satisfies them. Many will be free to pursue occupations which are nonrepetitive and require a sense of art and beauty. In the overall space colonies there may be colonies of artists and writers.
Some of these people will form specialized communities and will develop (or bring with them from Earth) their own characteristic ideas of how life should be lived, how a community should be organized. On Earth it is difficult for these people to form new nations or regions for themselves. Indian reservations, the demands of some black people that they be granted title to several states of the Union, the founding of the state of Israel—at the cost of displacing the native Palestinians—are Earthly problems. But in space it will become easy for ethnic or religious groups, and for many others as well, to set up their own colonies. We may see the return of the Cherokee or Arapaho nation—not necessarily with a revival of the culture of prairie, horse, and buffalo, but in the founding of self-governing communities which reflect the distinctly Arapaho or Cherokee customs and attitudes toward man and nature.
Those who wish to found experimental communities, to try new social forms and practices, will have the opportunity to strike out into the wilderness and establish their ideals in cities in space. This in the long run will be one of the most valuable results from space colonization: the new social or cultural forms people will develop. It will even be possible for individual families, or small groups of families, to go off on their own and homestead an asteroid.
For this venture, the people would need a small spacecraft. They would also need equipment for agriculture and mining, all of which would cost perhaps $50,000 or $100,000—the price of a house in many parts of the country. The risks would be low and if they started out from the colony and their engine quit a few days out, they could stop and fix it, or radio for the Interplanetary Survey to pick them up. They could navigate with no more than a telescope, a sextant, and a small computer. It would be easier than navigating across the Atlantic, with its storms and surprises.
There may be millions of mini-asteroids, 100 feet or less in diameter, each one capable of supporting a small group. The people who will favor them will be like Daniel Boone, who said that when he could see the smoke from his neighbor’s fire, it was time to be moving on. For rugged individualists, the Ayn Rand types who can’t stand the restraints of organized society, these mini-asteroids may prove a haven. Like the lonesome cowboys of the last century, they may provide much of the romance and adventure of the high frontier.
All this is far from the usual science-fiction scenario of settlers bravely battling the hostile conditions of Mars or Titan, being strongly marked by the struggle in the process. We are not talking here about grafting extra pairs of lungs into the colonists so that they can live on Mars or equipping them with infrared vision so they can see more clearly in the gloom of Venus. Instead, we are talking about taking people as they are and providing them with worlds made to their measure.
Planets, after all, are poor places to live. They have high gravity, which makes it hard to get down to or off from their surfaces. It is as if ocean-going ships had to start their journeys by climbing out of a whirlpool. The gravity of planets also interferes with heavy construction and getting around. Planets usually have harsh weather, or weather which at the least is changeable and often unpleasant, and they are subject to extremes of temperature. Even if they are small and quiescent, like the moon, they still have day-night cycles which limit the usefulness of solar power, as well as mountains or valleys which as often as not are in conveniently located. In view of this and their own unparalleled opportunities by contrast, the colonists may say: “The trouble with people who live on planets is they think small.”
Mars, the focus of so many hopeful dreams, might be bypassed. It will see its research centers for geology and other studies, but it appears to have few resources which cannot be had elsewhere. Even if it did, its gravity would make it costly to lift them out. Its atmosphere is just thick enough to prevent the use of a mass-driver. Yet the atmosphere is too thin to screen the solar ultraviolet or permit the use of aircraft for transportation. Mars of the great volcanoes, Mars of the deserts, of the frosty nights and the whistling winds in the canyons—if it is to be colonized, it will be done as an afterthought in the history of the human reach into space. It may remain a vast dry land, far from the major centers of commerce or population, thinly populated and of interest mainly to the people that live there. Mars may be the Australia of future centuries.