Colonies in Space:
Chapter 8 – The Highest Home Chapter 8 – The Highest Home
Colonies in Space
by T. A. Heppenheimer
Copyright 1977, 2007 by T. A. Heppenheimer, reproduced with permission
Chapter 8 – The Highest Home
Who will the space colonists be? What kind of work will they do and what skills will they need? To begin with, it is fairly certain that the principal types of jobs in the colony will be associated with large-scale construction or with running the colony’s agricultural and life-support systems. To fill these positions the government will seek people with experience or backgrounds in shipbuilding, heavy electrical construction, industrial construction, chemistry, experimental agriculture, and plant science. There will, of course, be a number of professional and administrative positions in the colony. But these will be filled largely from the pre-existing project staff, by people who already will be engaged in the Earth-based design and development work.
It seems likely that preference will be given to single workers, male and female, under age thirty. Married couples, both of whom are acceptable for employment opportunities in the colony, will be welcome to apply. Facilities for child care will initially be limited, so preference will no doubt at first be given to couples with no more than one child. (As the colony becomes more solidly established, of course, many more children will be welcome.) Affirmative action hiring procedures will be in force.
This last feature will have special significance in regard to the ratio of men to women in the colony. It will be important that from the start the work force has approximately equal numbers of men and women. A work force mostly of men would soon give rise to a most unpleasant social atmosphere, resembling, if not a boom town—with its attendant crime, prostitution, and inflation—then possibly a prison or an army camp. A sexually mixed work force will ensure the development of a more normal society. The colonists will meet, fall in and out of love, marry or divorce or live together and establish homes and families.
Legislation can help to foster a stable society in the space colony. Congress should pass a law providing that those who build the colony can attain the common goal of American society—a home owned free and clear; that is, a home in the colony itself.
These policies will encourage the settlement of space not by opportunists or speculators, but by homeowning families.
Now that we have a fairly good idea of who the space colonists will be, what will their surroundings be like? Gerard O’Neill had some comments on this, when he testified in Senate hearings on January 19, 1976. At the time, Senator Barry Goldwater was hosting hearings on the subject of space industry before the Senate Subcommittee on Aerospace Technology and National Needs. When Gerry O’Neill came to speak, the hearing room was jammed. As part of his remarks, O’Neill said:
It is natural for most people, and particularly for reporters and art directors, to become preoccupied with two features of orbital manufacturing. . . . One is, “Where is it [the space colony] going to go?” and the other is, “What is it going to look like?” I think the proper answer to the first question is “in an orbit high enough so that it almost never gets eclipsed,” and to the second, ”It will be a rotating pressure vessel, containing an atmosphere, with sunshine brought inside with mirrors.” Beyond that, any further detail is almost certain to be wrong. For that reason, among others, I think it’s unwise to get personally identified with particular designs. I’m for whatever works best, and it’s too soon yet to be sure what that will be.
It is true that it’s too early to say precisely where the colony will be or what it will look like. But it is not too early to say what would constitute a suitable location or shape.
For instance, a location on the moon is ruled out. Not only is there the problem of the two-week-long nights; the lunar gravity would make it very hard to build and launch power satellites. A low orbit around the earth like that of a space station could be tried. Such an orbit will stay in continual sunlight if it passes very nearly over the North and South poles and it is easy to reach it with rockets. But it is very difficult to transport lunar materials there, and it’s a bit more difficult than it should be to send the power satellites out to geosynchronous orbit. If the power satellites are built from Earth-launched components, it will be well into the next century before this will be a paying proposition.
What about geosynchronous orbit? It is no harder to reach than any location near the moon, it is almost never eclipsed, and a colony located there would have no problems delivering the power satellites. What’s more, it is already a much traveled orbit with satellites for communication and Earth observation. The founding of a colony there would be another instance in mankind’s long history of establishing cities upon the main routes of commerce.
But geosynch orbit has two disadvantages, one minor, one major. The minor one is that it lies directly in the middle of one of the Van Allen radiation belts. While it is not too hard to protect colonies and rocket craft from radiation, it’s better to avoid the Van Allen zones. The major problem is transportation of lunar materials there. Once launched to deep space by the mass-driver, the millions of tons of lunar ore cannot reach geosynch orbit easily. It takes slightly more than a mile per second of velocity change to get there. This is not hard for a rocket but to haul enough material to build the Great Pyramid of Cheops, it’s too much.
Beyond these obvious choices, things begin to be difficult. The reason is Earth’s gravity and lunar and solar pulls. These give disturbances to an orbit or, as an astronomer would call them, perturbations. The perturbations can be very important. For instance, some years ago there were some NASA officials who were interested in a space station to go into orbit around the moon. This was the LOSS, or Lunar Orbit Space Station. The interest declined abruptly when studies showed that after a few months it would crash into the moon.
In commenting on this, Robert Farquhar of NASA remarked in a report that the LOSS “would be a real loss.” He then went on to describe an alternative which he called HOSS, Halo Orbit Space Station. This idea was based on perturbations helping rather than hurting in some orbits.
One of these orbits would take the colony two weeks to go around the earth—half the period of the moon. If you consider only the perturbations of the moon, the orbit will continually shift away from the moon. This is also true when the effects of the sun’s perturbations are considered. Such an orbit is almost never eclipsed, and so is quite advantageous.
Prior to 1976, the most helpful perturbations, in the best-studied orbits, were thought to involve what are called libration points or Lagrangian points. In astronomy “libration” refers to a type of back-and-forth motion, like that of a pendulum, which bodies can undergo in the vicinity of these points. Libration points are locations where a spacecraft can be placed so it will always remain in the same position with respect to the earth and the moon.
Suppose the earth and moon were fixed in space and did not move. There would be a single libration point at the place where the gravity fields of the earth and moon cancel out. A body placed there would feel equal and opposite attractions from the earth and moon and stay fixed. If the body were moved a small amount, it would feel a slightly greater attraction from either the earth or moon. It would fall down, moving rapidly away from the libration point. Therefore, that point would be unstable.
But the earth and moon are in motion. It is the moon which does most of the moving. This means that in addition to the gravity of the earth and moon, account must be taken of the centrifugal force acting on a body in orbit. Libration points are the points where these three effects cancel out: the two gravity fields and the centrifugal force.
The French mathematician Lagrange showed in 1772 that there are five such points. Three of them lie on a line connecting the earth and moon; these are L1, L2, and L3. They are unstable; a body placed there and moved slightly will tend to move away, though it will not usually crash directly onto the earth or moon. The other two are L4 and L5. They lie at equal distances from Earth and the moon in the moon’s orbit, forming equilateral triangles with Earth and the moon. In the earth-moon system these points are stable.
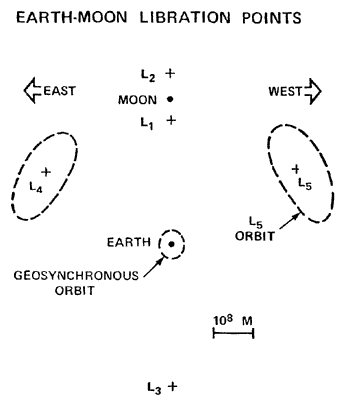
The situation, however, is more complex than this. The sun is in the picture and it disturbs the orbits of spacecraft and colonies. It turned out (from an extremely difficult calculation done only in 1968) that with the sun in the picture, a colony should be placed not directly at L4 or L5, but in an orbit around one of these points. The roughly bean-shaped or kidney-shaped orbit would keep the colony about 90,000 miles from its central libration point. It may seem curious to speak of an orbit around a point. Actually, the colony would be in orbit about the earth, but the simplest way to describe the orbit is from the point of view of an observer sitting always at the libration point.
It is also possible to have orbits around the other libration points. These orbits involve libration in two or three dimensions. Sitting on the far side of the moon and watching a spacecraft in orbit around L2, an observer would see the spacecraft make the same type of motion as a skywriting airplane making the letter 0.
Robert Farquhar calls such an orbit a halo orbit. It bears somewhat the same relation to the moon as does an angel’s halo to its head. The orbit is unstable, but Farquhar has shown that the solar perturbations on it are usually small and, by adroitly firing small rockets at the proper times, it is possible to stay in orbit. It was the basis for his proposal for the HOSS.
A halo orbit is not a very good location for a colony, because of the need to fire rockets regularly. But the L, and L2 points are very good locales for two of the major systems which support the transport of lunar material. L, is a good spot for the lunar power satellite to beam down the power to the lunar base. The powersat will follow a small halo orbit, firing small jets occasionally to stay on location. Also, the region around L2 is the right place for the mass-catcher. If the lunar base is on the great plains of the near side, then the material launched from the mass-driver can easily fly near L2.
So it was proposed that the colonies be near L4 or L5. The mass-catcher was to be near L2, and would shuttle between there and the colony. The lunar powersat would be at L1. (To date, no use has been found for L3.)
In the summer of 1976, though, several new studies were conducted which indicated that L5 was not the best location for the colony. One question considered involved the transport of lunar material from L2 to the colony. It turned out that with the colony near L5, this transfer would need a velocity change of over 1400 feet per second. So there was interest in finding a colony site which could be reached more easily. This problem was studied by the simple method of mathematically letting a catcher depart L2 and following it to see where it would go.
The resulting computer solution showed it would go quite close to a stable orbit around Earth with a period of two weeks. Further, it was found that if the colony were located in 110 such an orbit, it could be reached from L, with velocity change as low as 30 feet per second.
When it was also found to be easier to reach such an orbit from Earth than to reach L5, and also easier to deliver powersats from the orbit to geosynch, that clinched it. The colony will be in orbit some 200,000 miles from Earth at its farthest point and 100,000 miles at its closest point, taking a bit less than two weeks for each revolution.
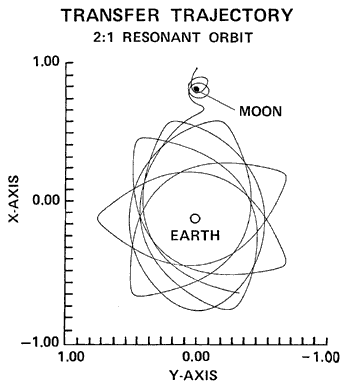
Once a locale is chosen for the colony, the next thing is to consider how it may look. On Earth a home or apartment can have almost any shape desired, but in space only a few standard shapes are possible. These shapes, in turn, are dictated by the needs which the colony must meet.
The first of these is gravity. The colony must rotate to provide artificial gravity, in the sense of a “down” toward which things fall. This is not real gravity, of course, but is centrifugal force. Like many other artificial things, artificial gravity will not be a precisely identical substitute for the real thing. It will be good enough, but its artificiality involves some side effects which can cause problems.
The colonists will be Earth people whose bodies are accustomed to the sensation of gravity. There is still not a complete understanding of what happens in its absence, but what is known about weightlessness is not completely reassuring. [Footnote by author: A word is in order here about the meaning of “weightlessness” or “absence of gravity.” It does not mean a part of the Universe where there literally are no gravity fields. The law of gravity is very hard to evade. “Weightlessness” means that an object is moving freely in response to whatever gravity 112 fields are present. If it ceases to move freely. whether by rocket thrust or by standing on solid ground. there is weight.]
Most of what we know about weightlessness comes from the experiences of the Apollo and Skylab astronauts. Shortly after reaching orbit, they often suffered from motion sickness. In Skylab, the motion sickness was especially bad, perhaps because of the increased room to move around in the rather large spacecraft. Fortunately, with the help of medication, the astronauts got over their spacesickness in a few days. After that, they had a greater tolerance than usual for motions that would ordinarily have made them want to reach for the little white bag.
The Skylab astronauts were able to live comfortably in zero gravity for the rest of their flights. However, after they got over their motion sickness, their red blood cell counts began to drop. Apparently there was a falloff in the production of red blood cells. Fortunately, after about a month in space, this loss of red cells leveled off, thereafter beginning to rise again on the longer flights. There was no increase in the body’s destruction of these vital cells, but only an interruption in their production. By the end of the 84-day third mission, the astronauts’ red-cell counts were nearly back to normal. Nor were changes observed in the immune response—the body’s ability to fight disease.
There was one medical problem which did not level off and improve even on the longest Skylab flight—the loss of calcium from bones. The bones of the human body react to weightlessness the same way they react to prolonged rest in bed: by losing calcium at about .5 percent per month. This loss was continual throughout even the longest Skylab mission. The Skylab astronauts also lost nitrogen and phosphorus.
The calcium loss is serious because it can weaken bones and make them more prone to fracture. This problem still has not been solved. Possibly it can be controlled by adding calcium to the diet and by having people in space perform exercises which not only give the muscles a workout but which also put stress on the bones.
The loss of calcium will not be a problem for periods of a year or so in space. It will be quite possible for crews to spend tours of duty in a weightless construction shack and very likely they can exercise or receive medical treatment to stay weightless for much longer times. But this can only be a temporary expedient. The colonists will certainly need to have weight.
It is desirable to make the colony as comfortable and Earthlike as possible. Space colony designers have tended to agree that the colony should provide normal Earth gravity, one g. We could consider lower levels, but we have no experience with living in levels of gravity between zero g and one g. The experience of zero g involves rather complex medical effects and clearly it is best to be cautious, to make no unneeded changes in the lives of the colonists.
The gravity will not be precisely the same everywhere in the colony. It will diminish toward the center. In a two-story home in the colony, gravity will be about .5 percent weaker on the second story than on the first. A 200-pound man would lose a pound just by climbing up the stairs. So it probably will be a popular thing in such houses to put the bathroom scale on the second floor. Even more weight loss will be possible by terracing the apartments with the topmost terraces 200 feet above the bottom. Then the variation in weight will be as much as 6 percent. For a number of reasons, the top terraces will be the most desirable real estate; but they likely will be most desirable to people on a diet.
However, there is a side effect to the artificial gravity which must be carefully controlled. This is the Coriolis acceleration, which is a property of all rotating environments. It is a tendency to change speed as you go inward or outward from the axis of a rotating object. On Earth it is the Coriolis acceleration which makes hurricanes swirl to the east in the northern hemisphere, to the west in the southern. It tends to make water draining from a bathtub swirl to the right in the northern hemisphere, to the left in the southern; but the bathtub must be kept very still for this to happen.
In a rotating colony, the Coriolis acceleration would have other noticeable effects. In a baseball game a batter will sometimes hit a long line drive which curves foul. This is because of the wind, but in a colony the same thing could often happen because of the Coriolis acceleration. Its tendency to change the direction of moving objects could produce other curious results. A colonist might be watering his lawn from a hose and see the stream curve over onto his neighbor’s barbecue. Or he might be taking a shower and the spray from the showerhead would curve onto the floor. If the rotation is fast enough and the rotating area small enough, even worse things might happen. A man might go to the bathroom to answer nature’s call and miss the toilet.
These rather unusual events would not really happen in any colony of reasonable size and rotation rate. But it is the Coriolis acceleration which gives rise to dizziness and motion sickness when a person is rotating rapidly. To avoid this, it is necessary to limit the allowable rotation rate.
At first, Gerry O’Neill believed the limit would be three rpm. However, colonists will be going to work in zero g, commuting back and forth between free space and their rotating home. While it is quite possible to become accustomed to continuous living at three rpm, it is likely that people would suffer from motion sickness if they had to go back and forth twice a day between such conditions and weightlessness. The 1975 Summer Study finally decided that the allowable rotation rate would be one rpm.
When you know the allowed rotation rate and the required level of artificial gravity, it is possible to determine the required size for a colony. When O’Neill thought the colony should give 1 g at 3 rpm, it would have a diameter of about 600 feet. To house 10,000 colonists with a reasonable amount of room for everyone, the colony would have to be a mile long. This was O’Neill’s cylindrical design for a colony, with mirrors to reflect in sunlight, the design he had discussed in his original article in Physics Today.
However, there is no requirement that a colony be built in the shape of a cylinder. The only requirement is that it be a simple shape which can hold an atmosphere. The colony in space is a sort of balloon or inner tube, with air inside under pressure, and to keep its weight down, its shape has to be similar to the shape of a balloon or tire, as we are familiar with them.
Before settling on a shape for a colony, there is one other criterion to be met. The selected shape should give colonists, living on the inside, a sense of spaciousness. They should be able to look around and see not only the buildings immediately near them but also long distances hundreds of feet away. They should be able to walk outside of a building and feel they are out of doors, not merely that they have gone from a small room into a larger one. It will be even better if the colony shape allows not only long lines of sight, but also keeps some parts of the interior out of sight. These unseen parts would be either around the bend or else at a fair distance. This is important because people do not enjoy living in an area which they can take in completely with one glance. They need to be able to get a change of scenery.
We know from experience on Earth that if people are in a large enclosure with a roof high overhead, they regard themselves if they were outdoors and not necessarily in a large room. Baseball fans in the Astrodome and in other domed stadiums are not aware of the roof overhead unless a batter hits a pop-up. In San Francisco and Atlanta are the Hyatt House Regency hotels; these feature lobbies with roofs 200 feet high. It is difficult to be aware of the roof since there are plants and people at eye level.
While with the 1975 Summer Study on space colonization, I had a different demonstration that a very high roof is not really a roof at all. At Ames Research Center where we 114 worked, there are several enormous hangars built in the 1930s to house dirigibles. Their roofs are 100 feet or more above the floor. Within them are small offices, used by some of the people who work there. The offices are simple affairs of plywood nailed together. They have four walls, a desk, a telephone—and a plywood roof. The hangar roof does not seem like a real roof; for the people to feel that they really are inside an office, they have to have a roof directly over their heads.
The need for spaciousness rules out some designs that otherwise would be quite appealing. For instance, a colony could be built like a space station, from a lot of small elements arranged in a ring. Such a colony would be easy to build, but from the inside it would look like what it is: a collection of tin cans.
When our 1975 Summer Study got under way in June, we expected our colony design would look pretty much like O’Neill’s cylinders. Thus it was a matter of considerable surprise when Wink Winkler, an undergraduate who was studying for admission to medical school, proposed that the proper rotation rate would be not three rpm but one rpm. This meant the whole colony design would have to change. When Winkler decided this in July, O’Neill was about to go to Washington to meet with Congressman Morris Udall and testify before the Congressional hearings. He did not exactly relish having to redesign his colony, especially on such short notice. So he sat down with Winkler and suggested a compromise: “Couldn’t we do it at two rpm?” Winkler didn’t say no and that was enough for O’Neill.
At first he thought of reviving an old idea, the “Bernal Sphere.” In 1929 J. D. Bernal brought out an extraordinary book of scientific predictions, The World, the Flesh, and the Devil. In that book he gave the first description of the cosmic-ark method of interstellar flight. In this a self-contained spaceship would provide for generation after generation of people to grow and reproduce till the remote descendants would reach the destination star. The starship, he said, should be a sphere.
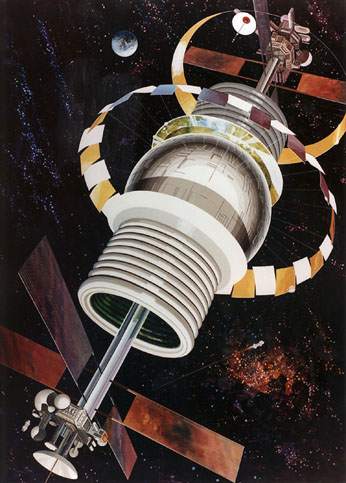
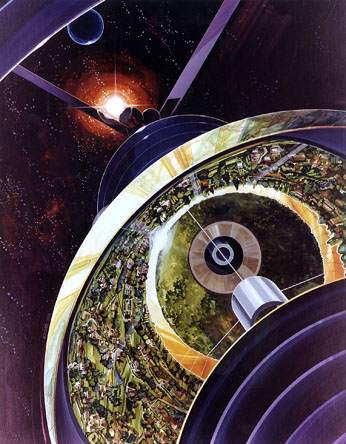
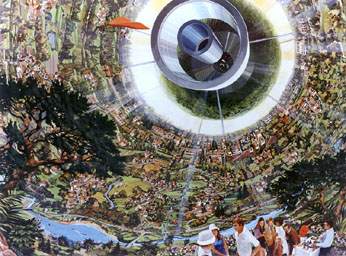
O’Neill adopted Bernal’s ideas to furnish a design for a colony. But he preferred a cylinder to a sphere; so he settled at last on a rather stubby cylinder, 1500 feet across. He christened it “Sunflower”: “Its petal-shaped mirrors, its tracking of the sun, its reliance on solar energy, and its property of being a warm habitat for life in the cold of space, all suggest the name.” It was this design which O’Neill proceeded to describe at the Washington hearings:
It allows for natural sunshine, a hillside terraced environment, considerable bodies of water for swimming and boating, and an overall population density characteristic of some quite attractive modern communities in the U.S. and in southern France. It is startling to realize that even the first-model space community could have a population of 10,000 people, and that its circumference could be more than one mile. From the valley area, where streams could flow, a ten-minute walk could bring a resident up the hill to a region of much-reduced gravity, where human-powered flight would be easy, sports and ballet could take on a new dimension, and weight would almost disappear. It seems almost a certainty that at such a level a person with a serious heart condition could live far longer than on Earth, and that low gravity could greatly ease many of the health problems of advancing age.
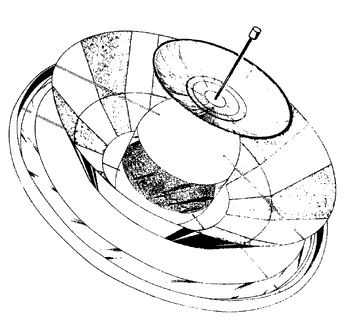
Upon O’Neill’s return, Wink was outraged that his recommendation of a one rpm limit should be treated so lightly. His careful research eventually convinced nearly everyone that the colony really should spin at one rpm.
A few evenings later about a dozen of the Summer Study people jammed into the apartment of Allan Russell, another Summer Study participant, to design a colony which would give one g at one rpm. Our architects were there as well as our structural designers and a number of others. The session lasted well into the evening. By the time we called it a night, we had the basic outlines of what would become known as the Stanford torus.
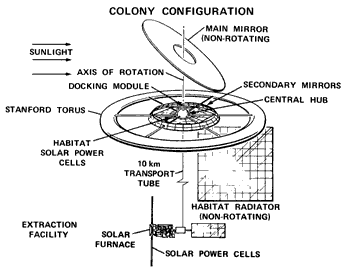
The spin rate and required gravity meant the colony would need a diameter of slightly over a mile. This immediately ruled out any sort of cylinder or sphere since it would then be too massive. Instead, we settled on a torus, an enormous inner-tube shape with the “tube” 400 feet wide and bent into a circle with a circumference of some 4 miles.
This design had many advantages. It was the lightest design yet studied. It offered the possibility of making effective use of interior space since there could be terraces for homes and agriculture. The colonists would have unobstructed lines of sight of half a mile and more; yet not all the colony would be open to view, since much of it would be concealed beyond the curve of the torus.
Once we settled on the Stanford torus, it was easy to design the rest of the colony. There would be a central hub. At its top would be a docking area, counter-rotated to stop spin, with ports for incoming spacecraft. The top of the central hub, which is 400 feet in diameter, would be the colony’s “north pole.”
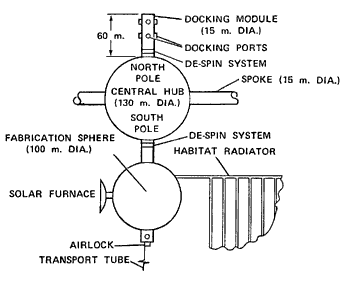
The hub will be more than the physical center of the colony. It will have recreation areas and low-g gymnasiums and swimming pools. The maximum gravity there will be only about one-sixteenth g; so there can be human-powered flight. The hub will not only be a recreation area, it will be the main crossroads of the colony. All people and freight entering will pass through it. It will be the central area where six large spokes converge, each with elevator shafts, power cables, and heat pipes. Through this hub thousands of commuters each day will pass on their way to and from work.
Directly beneath the “south pole” of the hub is a de-spin system to which a second sphere is attached. This will be one of the construction spheres from the shack. It will be the fabrication area where major components are put together for the power satellites. To one side of the sphere will be a solar furnace. To the other side, edge-on to the sun, will be the colony’s main radiator. This huge panel, a half-mile square, radiates the waste heat of the colony to space. Like the docking area at the north pole of the hub, the fabrication sphere and radiator do not rotate.
Below the fabrication sphere is an airlock and there is a long tubular passageway or transport tube. The tube runs to the second major industrial area, the extraction facility, where metals and other products are obtained from lunar material. Here is where the mass-catchers dock when they come from the L2 libration point. The connecting tube resembles the pneumatic tubes used for mail service in some large buildings. A group of workers or a roll of sheet aluminum can go into a capsule; then air pressure sends the capsule along the tube in a minute or so. At the other end of the tube, air pressure brings the capsule to a gentle halt.
Directly above the central hub is a huge yet lightweight mirror over half a mile across. It floats above the colony with small rockets to keep its position properly adjusted. It is angled away from the sun to reflect sunlight down onto a double ring of secondary mirrors. These are mounted in rings around the hub, supported by the spokes, to reflect sunlight into the colony. The mirrors can be tipped or tilted to reflect sunlight away. This is done twice a day during the colony’s dusk and dawn.
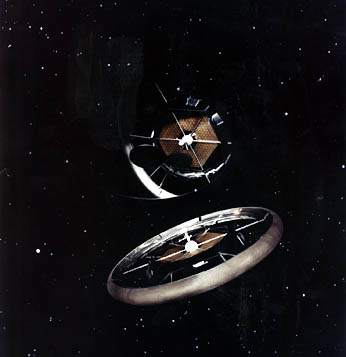
The spokes are fifty feet wide and go to the periphery of the colony. Not all the interior room is taken by the elevators, cables, and pipes which are so necessary to the colony. Together the spokes contain nearly as much room as in the Empire State Building. There are offices there and shops and laboratories for scientists. There, too, will be the University of Space.
The real life of the colony, however, is in the rim. There are terraced agricultural areas, homes, parks, and gardens. The inner one-third of the toroidal rim surface area consists of glass panels mounted on aluminum ribs to let in sunlight from the secondary mirrors. The remaining surface area is an aluminum hull of solid metal an inch thick, wrapped with cable.
Here is a city of 10,000, a small, bright island in the emptiness of space. Here will be the place where the human race first dimly glimpses its possibilities, its prospects, its future. It will be the true fulfillment of the dreams which once inspired a young and vigorous president in days when the world was newer and all things seemed possible:
“We choose to go to the Moon in this decade, and do the other things, not because they are easy but because they are hard. Because that goal will serve to organize and measure the best of our energies and skills. Because that challenge is one that we are willing to accept—one we are unwilling to postpone—and one which we intend to win.”