Colonies in Space:
Chapter 6 – The Moon-Miners Chapter 6 – The Moon-Miners
Colonies in Space
by T. A. Heppenheimer
Copyright 1977, 2007 by T. A. Heppenheimer, reproduced with permission
Chapter 6 – The Moon-Miners
The moon was formed four and a half billion years ago in scenes of titanic violence. It grew from the assembly of many much smaller bodies. At first, these bodies—each the size of Mount Everest, say—assembled through collisions, at speeds no greater perhaps than a fast freight train. But in time these collisions built a core, large enough to have noticeable gravity, and then the impacts grew in violence.
The colliding bodies were not usually of solid rock; often they were mere loose assemblages, themselves formed from many smaller bodies. Many, perhaps, were of a porous or fluffy structure. But of whatever nature, when they struck the growing moon they exploded with the energy of a hydrogen bomb. The explosions dug huge craters in the surface, blasting material back into space. But some of the material clung to the moon, and in this fashion it grew.
The moon in those days knew only bombardments, explosions, and the impacts of flying rocks. Great craters and mountain ranges formed, only to be buried, a million years later, by the unending sprays of rocks flung from other, newer craters.
For a hundred million years this went on. Then, at last, the bombardment began to diminish. The reservoir of orbiting bodies, from which the planets were built, simply was running out. But before it ended, the moon was rocked by a series of cataclysms vaster than any it had earlier known.
The planetesimals did not all stay merely as mountain-size bodies. Some had themselves begun to grow through collisions, just as the moon’s core had grown in its earliest days. Eventually, some grew to diameters of a hundred miles or more. It was these bodies which now proceeded to strike the moon.
The first of them struck in the southern latitudes of the lunar far side. Its energy was that of a million of the largest bombs ever built, but did not come from explosive material. Rather, it derived from the sheer speed of so huge a body. The impact sent seismic waves through the entire moon. Huge masses of rock coursed outward, burying portions of the lunar surface thousands of miles away. In the near vicinity of the impact—for several hundreds of miles around—the moonquakes were powerful enough to fracture the rock of the moon’s outer regions. Then titanic shock waves, radiating from the center, scooped up this thousand million million tons of rock and splashed it outward in an immense tidal wave, miles high.
When the shocks and flows of rock subsided, there was left a depression a thousand miles in diameter. Within it were three mountain ranges, concentric rings of rock, to mark where the tidal waves had at last spent their force. It was, perhaps, the greatest single feature ever formed upon the moon.
Yet it did not last. The final stages of lunar bombardment continued to build new craters, to erode existing mountains. When the bombardment finally ceased, the basin was heavily eroded, its rings of mountains worn almost beyond recognition; and so it remains to this day.
There were other great collisions, other surges of rock to dig immense pits upon the face of the moon. But at last the impacts ceased, for there were no more planetesimals. The moon rested.
Then slowly, over more hundreds of millions of years, heat built up inside the moon’s outer crust, as radioactive elements decayed. While the earth’s early oceans sparkled in the light of a younger sun, this heat melted rocks to lava. While blue-green algae formed matted accumulations in Earth’s shallow waters (not for three billion years would their remote descendants advance the few yards to the beaches themselves), this lava accumulated beneath the surface of the moon. Finally, within the deepest basins and craters, it welled up. For half a billion years this welling continued.
Sometimes the vulcanism produced only small, local features, like the curious domes in the region of the Marius Hills. Other times it sent vast sheets of lava flowing slowly across the surface. Then again, a pool of lava would drain off through an underground tube. When the tube was empty, it would in time cave in, resulting in one of the moon’s rills or canyons.
At last, the basins were filled in, and now even the vulcanism began to die. Yet there continued to be an infall of rocks from space. These were not planetesimals, now, but merely meteoroids, some larger than others. A few dug major craters, like Copernicus and Kepler. Most simply dug small pits and scattered small quantities of rock. In this fashion, the lunar soil became fractured and pulverized, to a depth of several feet.
For over three billion years there was no change. There was the endless cycle of searing, blazing sun and cold black night. More meteoroids fell, pulverizing the soil to deeper levels. Matter flowed out from the sun, and some of it implanted itself in the crushed stones of the lunar surface. Occasionally, a meteoroid larger than usual would fall, and would dig a crater. That was all.
These are the things the moon did not have. It had no water, not even water trapped in rocks. There was no air. Nor were there ores, or easily worked sources of metal. Only rocks, which might someday be made to yield up their content of aluminum and oxygen, iron and titanium—rocks, and the blazing, desiccating sun.
On the moon there was no life but on the earth, a quarter million miles away, an extensive community of life-forms was developing. In time, there arose upon the earth an animal with a rare sense of self-awareness. In time, some of its number came to look at the sky, and to learn to make tally-marks on bone. These saw the moon, and tallied now twenty-eight, now twenty-nine marks upon the bone. Slowly, dimly, the idea began to arise that there was order and regularity in nature.
Then one day, another object fell toward the lunar surface. Like many of the meteoroids which had fallen, it contained aluminum and oxygen. But unlike them, it was able to slow down so as to land gently on the surface. Within it were two living beings from Earth. They stepped forth onto the bright bleak surface and uncovered a plaque:
HERE MEN FROM PLANET EARTH
FIRST SET FOOT UPON THE MOON.
JULY, 1969 AD
WE CAME IN PEACE FOR ALL MANKIND
The first lunar visitors came as explorers and as scientists. They landed in their tiny cramped spacecraft and spent but a few hours before leaving. They set up instruments, made observations, collected samples. Some of them rode for several miles in lunar rover craft. When they departed, they left their footprints and the lower parts of their landing craft. If undisturbed by future visits, these will last for a hundred million years. Lunar conditions are that unchanging, lunar features are that permanent.
From what these first explorers have taught us, we now know much of the moon’s early history. We know more: we know the nature and composition of the moon’s rocks and soils. With this knowledge, we need not guess or wonder at what can be found there. We can use this knowledge as a foundation on which to build the colonies of space.
The fundamental idea of space colonization is the use of lunar and other extraterrestrial resources. With only the resources of Earth, there is no hope of building the colonies. With the resources of the moon, space colonies may soon become inevitable.
The lunar rocks are not rich ores, but they are adequate. They contain plagioclase and anorthosite, sources of aluminum. There is also ilmenite, for titanium and iron. Lunar rocks also contain silica—on Earth a useless waste product, but of value in space. They contain oxygen, too, chemically bound to the metals and the silicon. With the solar energy of space, it becomes possible to break down these rocks, to liberate their metals and their oxygen.
To build the colony, about 1 million tons of this rock must be shipped each year from the moon out into space. The task will be the project of a group of perhaps 100 people: the moon-miners. They will be the next group to go to the moon, now that the explorers have left it.
Their task will be straightforward: to gather the necessary lunar material and to launch it into space where it can be caught. To do the first, they will have bulldozers and power shovels built to operate in the lunar vacuum. To do the second, they will build a device called a mass-driver.
Their lives will be the harsh lives of workers at a remote outpost. They will be on the moon for limited periods—a year perhaps or eighteen months—and they will welcome the spacecraft which come to take them back. The work will often be monotonous and routine, especially after the initial construction is finished. For all that they will share the camaraderie that comes with being one of a few selected for important work. For it is on their work, more so than most, that the success of the colonization effort will rest.
One might ask whether it might be preferable to build a colony on the moon—a large comfortable place which would be more or less self-sufficient. Certainly something very much like this may develop in time. Over many decades large numbers of people may come to live on the moon as lunar operations expand.
But there are a number of reasons why that old science-fiction dream, cities on the moon, may be very slow in realization. For most purposes, free space is a better location than the moon for the building of a colony. To reach the moon, it is necessary to use rockets and supply their propellant. Not only is a colony in free space reached much more readily, but also it is much easier to bring large bulky items there. A colony can build huge, fragile power satellites and send them to another orbit; powersats could never be launched fully assembled from the lunar surface. The moon’s gravity also interferes with the erection of immense but delicate solar mirrors or radiators, which are much easier to build in space. Finally, life on the moon will forever be influenced, and in many ways limited, by the two-week day-night cycle. This cycle may make it very difficult to grow crops. For all these reasons, the population in space colonies may be expected to grow much faster than the population on the moon. For a long time, then, the lunar activities will be carried out at a simple outpost or base, heavily dependent upon regular rocket flights from Earth.
Although space colonists will outnumber the moon-miners, there will be moon-miners before there will be space colonists. One of the early tasks in the space colonization effort will be the buildup of the lunar base. Only when it is functioning properly will it be possible to begin constructing the colony.
The first lunar flight in this effort will serve to found the base, to establish its location. In time a fair-sized city may grow up there, but to begin there will only be a collection of payload canisters from the rocket. This first flight will not land a fully assembled base, ready to support major operations. It will carry a small initial group of people together with what they will need to live on the moon for a while. Their task will be to survive, and begin the work which later flights will bring to completion.
This first flight will carry the initial items of earth-moving equipment. Among the most necessary of these will be a soil-blower, resembling a snow-blower, designed to scoop up loose soil and deposit it in a pile off to one side. There will be a tractor to pull heavy loads across the surface.
It is quite impossible to run this equipment with ordinary diesel engines. A diesel could be made to work in the lunar vacuum by supplying oxygen from a tank to the carburetor. But the exhaust from the diesel would prove difficult to restore chemically to its original state as diesel fuel. All of the lunar engines will burn hydrogen since hydrogen burns to produce water vapor, which is easily electrolyzed, recycling the exhaust to give back both hydrogen and oxygen.
For high efficiency the fuels should not be burned in anything resembling a diesel engine. Instead, they will be combined directly to produce electricity in fuel cells. These have been used since 1965 to produce power aboard manned spacecraft and they will serve as well at the lunar base. The first flight will also carry stores of hydrogen and oxygen for fuel.
One of the payload canisters will contain a most important item, to be handled with great care: the interim nuclear power plant. To bring a nuclear plant to the sunny moon may be seen by some as akin to bringing oil to the Arabs. There will be much interest in solar power for the lunar base but on the moon, the sun shines only two weeks of the month and nuclear power is available round the calendar.
The interim plant will be a simple affair of 100 tons mass. Even in the moon’s low gravity, it will still weigh sixteen tons; so the tractor will be needed for its installation. With pulleys and winches, the work crew will lower it to the surface setting it on a wheeled chassis. Then the tractor will haul it a mile or so away. Other work crews will use the soil-blower to pile mounds of earth around its core for protection against radiation. They will set up a large radiator which gets rid of waste heat so that the reactor can generate electricity properly. They also will install electric power lines to bring the current to the base.
For living quarters, some of the payload canisters will be fitted out as a type of lunar quonset hut. The huts will be comfortable enough, the way a Holiday Inn is comfortable, but they will hardly cause the moon-miners to fondly remember their times there. These will also be detached from the landing craft and hauled to their locations. The work crews will then proceed to cover the huts with several feet of lunar soil, using the soil-blower. This will protect them against cosmic rays and solar flares.
The covering of soil will make it easier to keep the internal temperatures comfortable. In space temperature control is mostly a matter of exposing black- or white-painted surfaces to the sun, but on the moon it is made more complicated by the day-night cycle. A covering of soil will help protect the huts from extremes of temperatures. It is easier to heat a room than to cool it and this is particularly true in space. So the moon-miners quite likely will set up sunscreens to keep their huts in permanent shadow. The soil covering then will act as insulation and heat will leak out only slowly. The heat from the miners’ bodies and from equipment within the huts may act to maintain comfortable temperatures inside.
With electrolyzers to produce hydrogen and oxygen, powered by the nuclear plant, the beginnings of the lunar base will be complete. The population will eat the ordinary food of astronauts: freeze-dried meats and vegetables, Tang, Pillsbury food sticks, all reconstituted with water produced by the fuel cells.
The base will then be ready for expansion, prepared to grow into the major facility upon which so much of space colonization will rest. The growth will come with the arrival of other rocket craft, perhaps twenty in all, over a period of three or four years. Some of these will carry return rocket stages to rotate crews back to Earth. Most, however, will bring more moon-miners, more quonset huts, more equipment of all types, more food and fuel. They also will bring components of the two major lunar systems: the main nuclear plant and the mass-driver.
The main plant will generate 200,000 kilowatts of power, enough to supply a city of 100,000. Some of this will serve to run the base. There will be no lack of electrolyzers, gas liquefiers, communications gear and hot-water heaters. But most of the power will serve to run the mass-driver.
The mass-driver will be an electrically driven launcher, a sort of electromagnetic catapult. It will accelerate masses of lunar material to escape velocity, 1.5 miles per second. These masses are to be launched, one or two per second, day in and day out, indefinitely. They will fly out into space, curving in the moon’s gravity and slowing down as they go outward. Two days after launch they will reach the catching point, 40,000 miles above the lunar far side. There they will be intercepted.
Nothing like the mass-driver has yet been built. Nevertheless, it rests upon such long-understood principles, such well-established engineering designs, that already we can describe it in great detail. These designs have been prepared for use in high-speed trains.
For a number of years, there has been considerable interest in building railroads which could compete for speed with airliners. In recent years, most attention has been directed to magnetic levitation and to propulsion by linear electric motors. It is this technology which will serve to build the mass-driver.
Such wheelless railroads (a term which may become as quaint as “horseless carriages”) now appear to represent a transportation development as important as the auto or airplane. The first commercial trains of this type may be built in Japan. The Japanese National Railways were the first to demonstrate an experimental railroad of this new type, on June 25, 1972—a date which in the history of transport may one day rival the Wright Brothers’ December 17, 1903, or Robert Goddard’s March 16, 1926.
In such a train, as well as in the mass-driver, superconducting magnets lift or levitate the vehicle above a track of aluminum. The magnets produce no lift when the vehicle is at rest. However, at speeds of only twenty miles per hour the metal of the track no longer allows magnetic lines of force to penetrate freely. The lines of force tend to be rejected from the metal, thus generating lift. At sixty miles per hour this effect reaches nearly full strength; the lines of force are almost entirely rejected, and lift approaches its maximum. At still higher speeds this magnetic repulsion changes little, but now there is a new effect. The repulsive levitation produces drag, but as the velocity increases the drag diminishes. This is quite in contrast to the situation pertaining to ordinary trains or airplanes, whose drag increases with increasing speed.
There are a number of ways to propel such a train. One of the most promising is the linear synchronous motor, which is to act on a powerful magnet aboard the train. A synchronous motor is a type of electric motor, and like all such motors it spins round, to produce torque. But such motors can be “unwrapped,” or built in a straight-line design, along a track. Such a linear motor then produces, not torque, but acceleration along a straight line. A train, which is magnetically levitated and driven by linear synchronous motor, can be likened to a magnetic surfboard. The surfboard rides the forward slope of a traveling magnetic wave whose speed of travel and height are continually adjusted to keep the surfboard in smooth motion. The adjustments are carried out automatically, and give the description “synchronous” to the motor.
These developments will be applied to the mass-driver. At the cost of delivering some 20,000 tons to the moon for the lunar base, it will be possible to ship some 1,000 times this mass to the colony as unprocessed lunar material. The cost of the transport will be some fifty cents per pound and may be less. No method employing rockets can compete with this system, any moi than air freight can compete with the cost of moving bulk goods by pipeline.
Both the mass-driver and the main nuclear plant will be shipped from Earth in modules of some 150 tons each. This is dictated by the lift capacities of the launch vehicles to be used. For the mass-driver most of the 150-ton packages will be loads of rail segments, or of sections for the trackside linear synchronous motor. The mass-driver will be built much as if it were a railway. There will not be spikes to pound or ties to lay, but there will be fabricated 100-foot lengths of aluminum track to lay, each weighing 400 pounds in the lunar gravity. These will be set in place upon the ground and welded together. Optical targets resembling bull’s-eyes will be placed atop the track. When viewed with a telescope, sighting along the track, it will be possible to adjust the track alignment to ensure adequate straightness.
The main power plant will require much greater effort. Its sections, delivered in small groups aboard each new rocket to land, will be quite bulky as well as delicate. There will be generators, turbines, and systems for circulating water within the reactor. There will also be large radiator panels, monitoring instruments, safety systems, and the core of the main reactor itself. The total system will be some 10,000 tons in mass. Slowly, carefully, the assembly will proceed, with tractors and other equipment. These will be powered by fuel cells, the fuel for which will be produced with power from the interim plant.
In time, the last length of track will be laid, the last components of the power plant assembled. The major phases of lunar base construction will be at an end, and the moon-miners will be ready to supply materials for the colony.
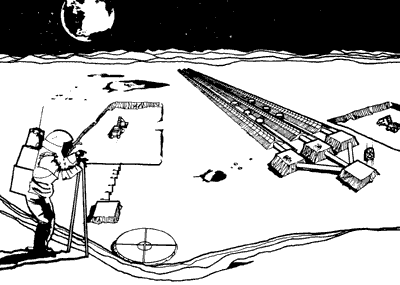
The lunar mining and transportation operation. At left, scoopers pick up loose soil from a pit, for delivery to the station at the right. There, the material is packaged into payloads and loaded aboard payload carriers (“buckets”) to be accelerated for flight. The triple mass-driver provides this acceleration (Courtesy NASA).
Strictly speaking, it is not correct to call them miners, for they will not burrow beneath the surface. For the most part they will simply scoop up loose material lying at the surface, the fragmented debris of 3 billion years of meteoroid bombardment. They may have equipment for prospecting. This equipment, mounted on roving vehicles, will identify rock types by their response to electric or magnetic fields. But even the richest deposits will have only two or three times the metal content of ordinary soil.
A million tons a year will be the goal, the quantity of material to be gathered and launched. That is equivalent to digging out twenty football fields to a depth of ten feet. This is work for only a single small power shovel operating continuously. After twenty years, this activity still will not produce a crater visible in the earth’s largest telescopes.
It is a curious thing, this view of humanity’s works from a cosmic perspective. Men fancy that they have transformed the earth, and perhaps they have. Yet from only a few hundred miles up, most works of civilization are invisible, or are difficult to distinguish from geological features. So much more difficult will it be to make a noticeable impact upon the moon.
Not for lack of effort will this be true, however. Once in full operation, shifts of moon-miners will be at work at all hours. In the glare of the sun, they will unroll small aluminum shades and go about their work. In the lunar night, the soft bluish glow of earthlight will shine on them.
The soil and small rocks gathered will be brought to the central station of the mass-driver. There they will be bound together in forty-pound packages, each surrounded by a fiberglass bag made from lunar silica. There will be a small processing plant to make the crude fiberglass and the wrappings directly from lunar soil.
The central station also is an automated facility for servicing the vehicles which ride the track and which carry the packages. These vehicles are the “buckets.” Each has a pair of superconducting magnets to support and guide the bucket along the track. It is these same magnets upon which the trackside linear synchronous motor sections will work to accelerate the bucket. There also is an array of mechanical restraints to hold the package during acceleration.
The superconducting magnets require the coldest substance known, liquid helium, which at -452° is only seven Fahrenheit degrees above absolute zero. When a bucket is in the central station, its main tank is filled with this frigid substance. During its last trip along the mass-driver, some of it will have boiled off. The gas passes around the outside of the magnets, to further insulate them, and runs into another tank aboard the bucket for storage. While still in the central station this tank is tapped and the helium drawn off to be reliquefied. A package of lunar material, in its fiberglass wrapping, is placed aboard and secured with four metal plates.
At this moment two or three dozen other buckets are in the central station, undergoing similar servicing. They all are guided on tracks, rolling on small wheels, so the central station actually functions as a railway switchyard. With precision timing each bucket is guided onto the main mass-driver track, like a car entering a freeway during rush hour. Once on this track the bucket quickly speeds up and levitates above the track. It is no longer supported by its wheels.
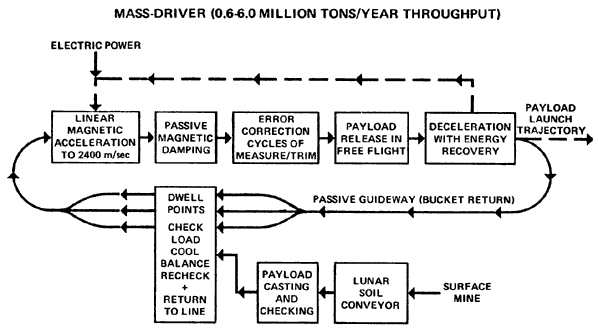
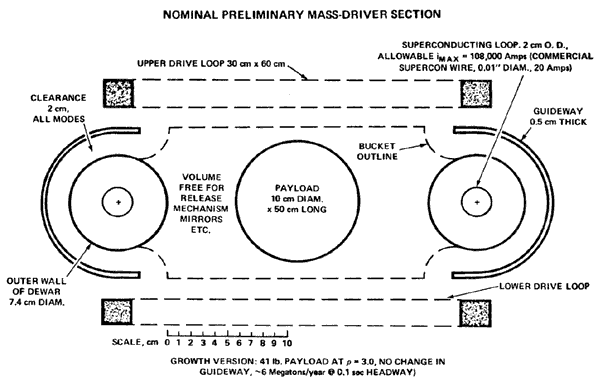
The initial acceleration lasts three seconds. In this time the bucket travels two miles. The acceleration is at 100 times Earth’s gravity, and the speed is carefully measured with laser instruments. Then the bucket is at lunar escape velocity. Next, the bucket traverses an accurately aligned section of track wherein no acceleration is applied. The accurately aligned section permits the bucket oscillations caused by bumpy acceleration and a slightly uneven track to die out, the way a car shaking on a rough road becomes steadier once it hits even pavement. This is known as “passive magnetic damping.”
The main restraining plates then drop away, and the package of lunar material is ready for release and for the start of its long flight. But it will not be released just yet.
Instead, the bucket and its payload fly out from the end of the track, in free space. They pass through a number of cycles of course adjustment, in each of which the bucket is tracked with laser beams so as to determine deviations from the planned trajectory. Then, magnetic coils apply trimming forces to the bucket, correcting for the errors. After ten to twenty such cycles of measurement, calculation, and trimming, the bucket and payload are within better than one part in a million of the planned velocity and position, and the payload is ready for launch.
At launch, while the bucket is in free flight, the last payload holds are retracted. The bucket, with the payload now floating inside, re-enters the track. The track then angles sharply downward, to snap the bucket away from the payload, a distance of six feet in 0.05 second (170 g’s of acceleration). The reason for this is that the payloads themselves are slightly magnetic.
Lunar soil contains some magnetic iron, in a very fine-grained form which is difficult to separate out. Moreover, most common minerals exhibit a weak form of magnetism known as paramagnetism. Both types of magnetism mean the payload motion will not be completely independent of the powerful magnets on the bucket. These magnets may disturb the payloads’ flight by only a few inches per second if the bucket is snapped away rapidly; yet even this error must be corrected.
So the payload passes through three more cycles of correction. The first of these involves a correcting coil at the start of flight, to take out most of the error introduced during snapout. The second and third cycles involve a slightly different method. At the correction stations, 2 and 100 miles downrange, electrons are sprayed on the payload, to charge it up to a good voltage—say, 100,000 volts. By laser tracking, required course adjustments are found. Then the payload passes between pairs of electrically charged plates, which adjust the motion in the same manner as electrons are controlled in a TV set. By the time all course corrections have been completed, the required launch direction, at a velocity of some 1.5 miles per second, is achieved to a precision of ten feet per hour in the velocity from side to side, or up and down.
The velocity itself, as well as its direction, may also be controlled to that accuracy, though it is more difficult to control the velocity accurately than to control its direction. But it turns out that this is not necessary because of a remarkable development in celestial mechanics.
By computing orbits to be followed by the payloads, it has been found that if they are launched from a suitable point on the lunar surface and aimed at a target, they will hit that target even if the launch velocity is slightly off. That is, the gravity of the earth and moon acts to focus the trajectories so they arrive at the target despite slight errors in velocity. The best such launch site is at 33.1° east longitude, near the craters Censorinus and Maskelyne. Then, the target can be chosen as a point in space 40,000 miles behind the moon, known as the L2 point. A catcher or target located there will stay on station, since there the gravity of the earth and moon is cancelled by the centrifugal force due to the orbital motion of the catcher.
After snapout, with the payload on its way, the bucket enters a section of track where it is decelerated. Powerful sections of linear synchronous motor slow it down, at 300 times the acceleration of Earth’s gravity, while recovering the energy of the bucket. When the bucket is below 100 miles per hour, it enters a banked and curved section of track. Now it will return along the backstretch of the mass-driver, to be guided to the switchyard and the central station in preparation for another launch.
Meanwhile, the payload flies onward, launched with astounding accuracy. If Garo Ypremian were to stand in the Oakland Raiders’ stadium, near San Francisco, and kick a field goal between the uprights of Robert F. Kennedy Stadium in Washington, D.C., that would be the accuracy of launch. If Nolan Ryan, across the bay in Candlestick Park, were to pitch a strike to the plate at the Big A in Anaheim, 400 miles south, it would be the same accuracy.
The flight is initially nearly flat, a low line drive slowly rising above the lunar surface. Not for 200 miles (2 minutes of flight time) is the payload high enough to clear the lunar mountains. This is why the lunar base and mass-driver must be located on one of the broad lunar plains which were filled with lava 3 billion years ago. Thereafter, the rise into space is increasingly swift. Two days after launch, the payload is 40,000 miles behind the moon and approaching the catcher.
The catcher is a huge self-propelled craft, 300 feet wide and a quarter-mile long. During the years of the lunar base buildup this catcher and its mate (there are two) will be assembled in Earth orbit and moved to deep space. The catchers double as space ore carriers, transporting hundreds of thousands of tons of lunar material to the site of the colony. While one is in transit, another is on station.
Each catcher has a large rotating conical bag of Kevlar fabric. Kevlar is used in bullet proof vests—a nine-ply layer will stop a .44 magnum shell fired point-blank. This strength is needed, for the payloads come flying in at 600 miles per hour. They resemble the cannonballs of eighteenth-century naval warfare and catching them will prove a difficult problem.
There is a grid of cables across the front of the catcher. The package of lunar material strikes this grid and breaks up. This releases a shower of small rocks and gritty sand which flies inward. The material strikes the sloping sides of the Kevlar bag, bounces a bit, and comes to rest. The rotating bag holds it in place through centrifugal force.
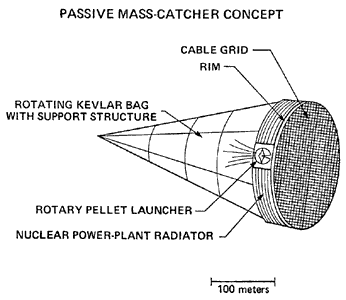
From a distance the catcher resembles a sawed-off dirigible. The size and shape are similar and the resemblance is heightened by the seemingly tiny propulsion units on board. Yet each of these is a rotating tube, 100 feet long. Spinning rapidly, these “rotary pellet launchers” eject small pellets of rock, giving thrust to keep the catcher on station.
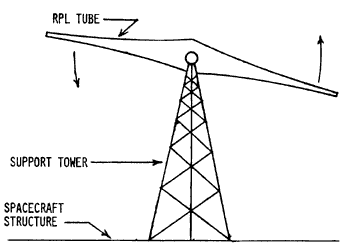
When the catcher is full it spins up its pellet launchers and slowly trundles off toward the colony site. It is now a true ore carrier, with loads and trip times quite like those of the supertankers of Earth’s oceans. Like these supertankers, it is run by a small specialized crew who endure weeks of boredom during the main portions of the long hauls. These routine trips, more than any other flights in the space colonization program, will resemble the long, boring hauls of science fiction.
From the cargoes of these flights, from the megatons of rock and soil so carefully launched and transported, the colony will grow.
There will be moon-miners before there are space colonists. But once the colony is well established, it will provide for an expansion of the lunar base by building a new power supply for it.
The colony will earn its economic keep by building power satellites. The first of these will be maneuvered toward the moon. Forty thousand miles above the near side, it will be stabilized in position, its power beam directed to the lunar base. There, solar power will at last replace nuclear power.
Nuclear plants will serve for the early years of the lunar base as a ready source of power. But it is no more desirable to rely on nuclear power for the long run upon the moon than upon the earth. Just as on the earth, solar power from a satellite will be the long-term supply which ensures the permanence of the lunar base.
Prior to the arrival of the power satellite, the moon-miners will prepare for it. They will build large trough-shaped reflectors of aluminum to concentrate and gather the microwaves to be beamed from space. Possibly they will build a small aluminum plant there to meet the needs of the lunar base and render themselves that much less dependent upon the earth.
They will not at first gather all the available microwave power since they will not need it. But as the years go by, they will build more and more trough reflectors. They will construct another mass-driver and yet another, to meet the growing needs of the space colony. As they expand, the power satellite will be there, providing the power they need.
Life at the lunar base will continue to be harsh. The moon-miners will continue to depend for many things, if not on the earth, on rocket flight from the space colonies. Not for a long time will they develop the comforts and conveniences of the colonies, and the moon-miners may continue to regard themselves as mere transient workers at an outpost.
For all this, during lunar night people will view the moon with telescopes and see something unusual there. In the extreme southern part of the Mare Tranquillitatis, there will be clusters of lights where before there have been no lights. There is a settlement on the moon.
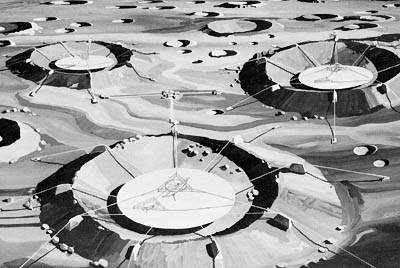